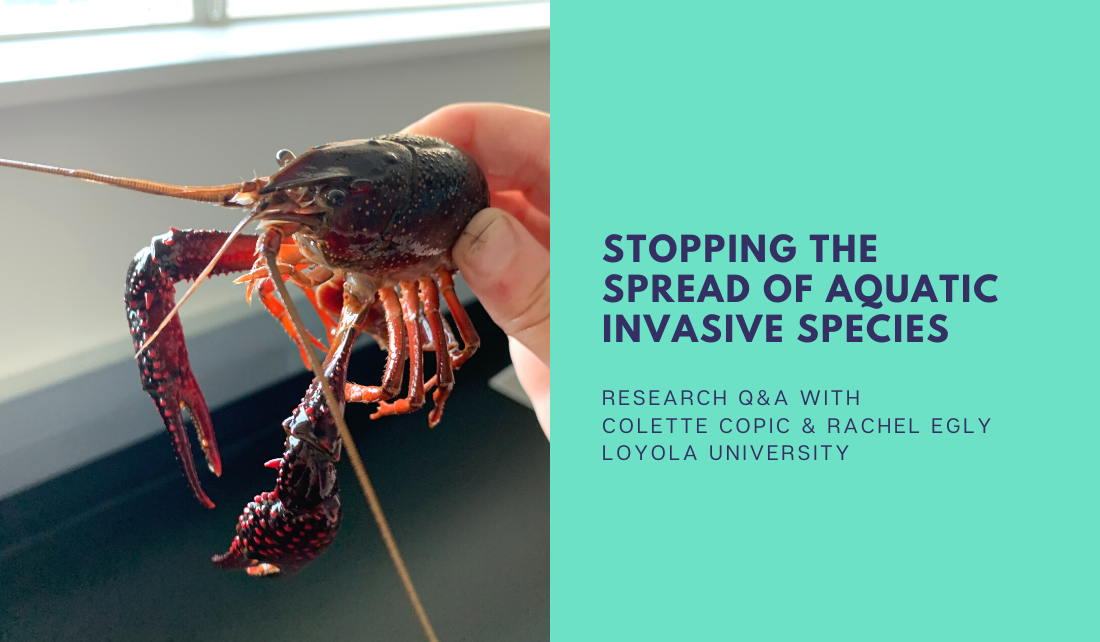
In this abbreviated interview, Colette Copic and Rachel Egly of the Keller Lab at Loyola University Chicago tell Illinois-Indiana Sea Grant Communications Associate Sarah Gediman about their research to contain invasive aquatic invertebrates. The team tested barriers on bloody red shrimp (Hemimysis anomala), zebra and quagga mussels (Dreissena spp.), rusty crayfish (Faxonius rusticus), red swamp crayfish (Procambarus clarkii), marbled crayfish (Procambarus virginalis), bladder snail (Physella acuta), Chinese Mystery Snail (Bellamya chinensis), Daphnia magna, and Hyalella azteca. While some of these species are not invasive, they served as substitutes for similar species that could become invasive.
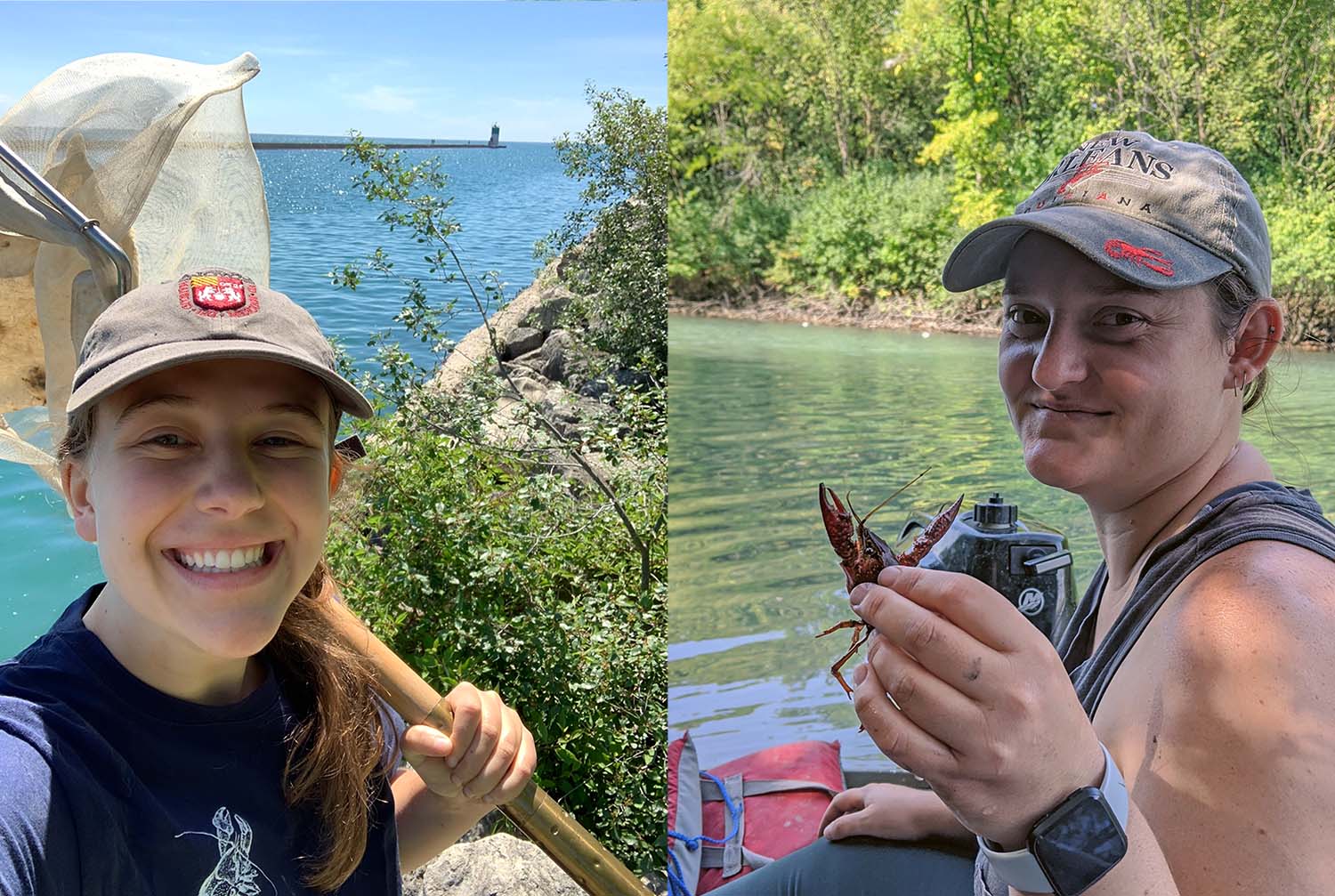
Colette Copic (left) and Rachel Egly (right). (Keller Lab)
How did you become interested in studying aquatic barriers?
Egly: We have worked with several aquatic invasive species, but we were inspired by the current electric barrier in the Chicago Sanitary and Ship Canal. So far, this barrier has succeeded in blocking Asian carp, which can disrupt entire ecosystems and economies, from entering Lake Michigan. While this has worked well for Asian carp, we aren’t sure how electric barriers affect other types of organisms.
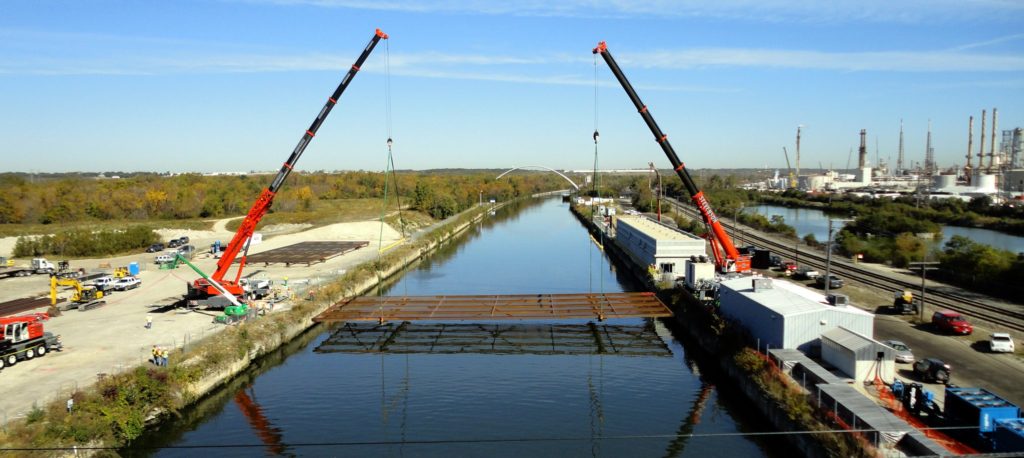
The first of three electric barriers in the Chicago Sanitary and Ship Canal was installed in 2002. (Sarah Gross / U.S. Army Corps of Engineers Chicago District)
Our lab was funded by Illinois-Indiana Sea Grant to look at how aquatic invasive invertebrates might respond to electric barriers and other methods of abating invasive species. I have been testing barriers using various strengths of electrical current, and Colette has been testing barriers made from carbon dioxide (CO2). We chose aquatic invasive species that have the potential to drastically change ecosystems should they become established populations.
Copic: Part of our motivation behind this project is that the scope is immense— canals are everywhere, and they aren’t going away anytime soon. We need them for trade, flooding management, and to be resilient to climate change, but every canal brings a free-flowing path for invasive species. If we can create canal management systems that allow ecosystem integrity, then this project has global implications. The question we want to answer is how we can have non-physical barriers in these canals so ships can pass through, but invasive species cannot.
We know electric barriers work, but what made you decide to test CO2 as a barrier?
Copic: The whole reason we’re thinking about this at all is that we know Asian carp avoid water with high CO2 concentrations in both the lab and the field. As I learned that, I thought, could this be a catch-all for other invasive species? Relative to other pesticides and biocides that we can use in water, CO2 is non-toxic and dissipates naturally. It won’t kill everything like a lot of other methods of keeping invasive species out, but it can immobilize and kill certain organisms, some of which are invasive.
How did you measure the efficacy of electric barrier and CO2 barrier techniques on your subject organisms?
Copic: I did two types of experiments. The first was in simple tanks to look at what behavioral effects carbon dioxide has on these creatures when they can’t escape. I tested their behavior on a range of carbon dioxide concentrations by observing the animals minute-by-minute in tanks with differing amounts of CO2. After recording the organisms, I got an idea of each species’ tolerance to CO2. Some become immobilized (wholly or partially), some are unaffected, and some die.
I also tested species that are mobile and can move across water to see if, given the choice, they would avoid high concentrations of carbon dioxide. I recorded where they were in the tank and saw that as levels of CO2 rise in one chamber, the animals will move to the chamber with lower levels of CO2.
Egly: Like Colette, I measured by behavioral analysis, with similar results. Here is a diagram of the lab-scale electric fields used to mimic the one in the Chicago Sanitary and Ship Canal. We put wires on either side of a large fish tank and ran electricity between one steel plate electrode and the other, which created an electric field.
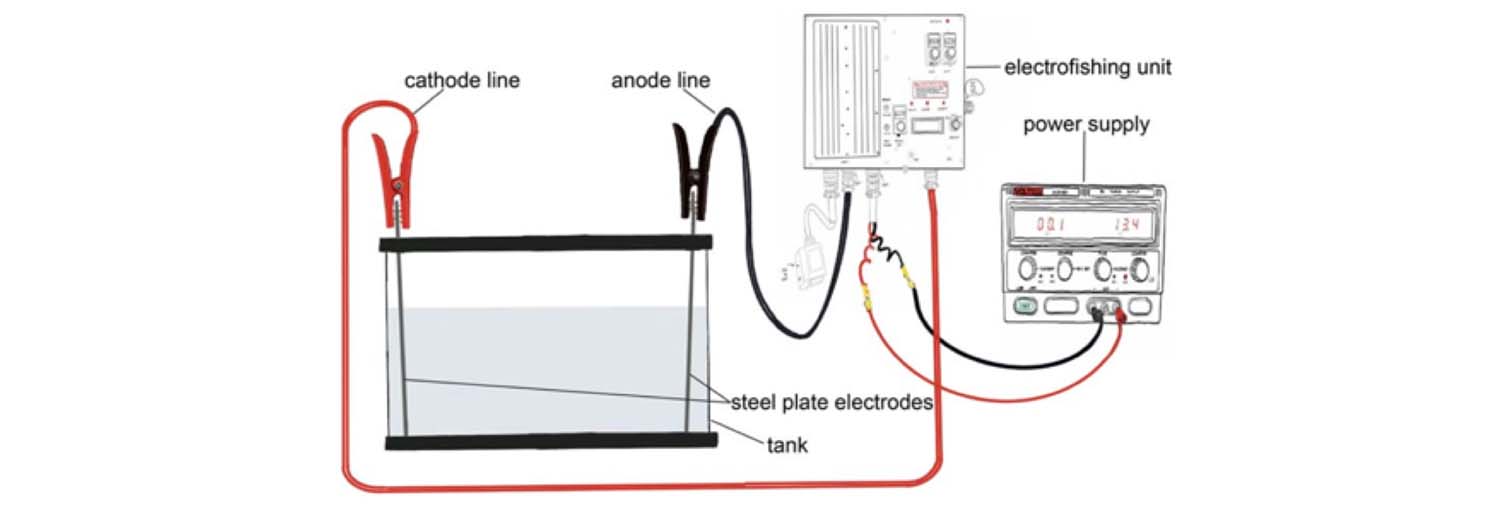
Diagram of experimental electric barrier setup, including power supply, electrofishing unit, tank, steel plate electrodes, and cathode and anode lines. (Keller Lab)
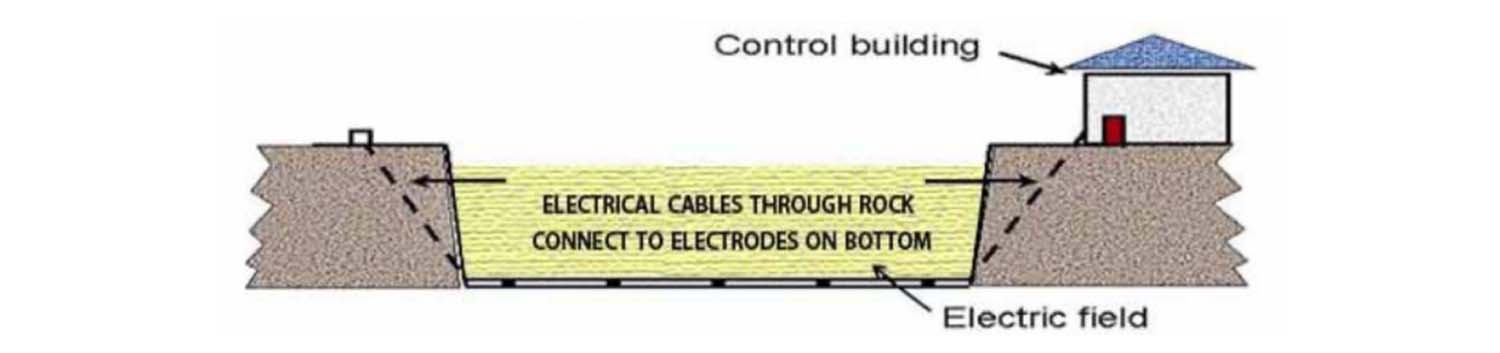
Diagram of electric barrier structure installed in the Chicago Sanitary and Ship Canal. (U.S. Army Corps of Engineers Chicago District)
What happens, ideally, when an organism comes into contact with CO2 or electrical currents?
Copic: I called the effect I saw narcotization because the CO2 was almost like a sedative. The crayfish would lose all muscle abilities and locomotive skills at higher CO2 concentrations. You could see they were experiencing stress as they got stiff, and some became completely frozen. I’d even poke them every five minutes and there would be no response. But then after just 10 minutes in fresh water without heightened CO2 concentrations, they were moving normally and it’s like it never happened.
Egly: I also found that even if the animals don’t exhibit a behavioral response such as lack of consciousness or motion, they still tend to orient themselves perpendicular to the electric barrier. So, we know that they’re feeling something, and they reduce their chance of being electrocuted by avoiding the barrier. We found that different species have different resistance to the electric barrier, so there is no single “ideal” strength of the electric field. The same is true for CO2 — different species react differently. Our results show that there is great promise for each of these types of barriers if the strength of each can be optimized.
What would these barrier systems look like in a real canal?
Egly: New electric barriers would look a lot like the current one in the Chicago Sanitary and Ship Canal, but with various amounts of electricity. In the existing structure, there are steel cables laid underneath the canal through which electricity is run to create a permeable division in the form of an electric field. This can be recreated in canals in many parts of the world.
Copic: Carbon dioxide really hasn’t been used much, so it’s tough to say. I’ve heard ideas about bubbling CO2 gas directly into strategic, small areas of the canal almost like a curtain. I’ve also heard the idea of having tanks on the side of canals, pumping CO2 and water inside, and releasing the mixture into canals when there is a high risk of species transmission. For example, when the shipping locks are open, or when electric barriers fail because they’re susceptible to power outages and might need maintenance.
You can learn more about the exciting research that Colette and Rachel are conducting in the Keller Lab at Loyola University Chicago by following @Craychel_Egly and @ColetteCopic on Twitter.
Illinois-Indiana Sea Grant is a part of University of Illinois Extension and Purdue Extension.